Chest wall resection and reconstruction
Introduction
Chest wall resection is indicated for the treatment of most primary chest wall tumors, some locally-invasive neoplasms and, occasionally, to control infection, ulceration or pain from malignancy or radiation. A surgeon’s primary goals in any chest wall resection and reconstruction should be to remove the lesion of interest, obliterate dead space, restore chest wall rigidity, preserve ventilatory mechanics, and protect intrathoracic organs, all while minimizing deformity. Although some basic tenets must be followed, innovative techniques have been described and may change the way that chest wall resection and reconstruction is performed in the future.
The work-up of a patient being considered for a chest wall resection should start with a thorough history and physical and the review of the patient’s computed tomography (CT) scan or magnetic resonance imaging (MRI). Points of interest are the location of the lesion, its size, rate of growth, attachment to surrounding tissues and presence of critical adjacent structures. Patients with benign-appearing or small (<4 cm) lesions should undergo excisional biopsy, while those with larger lesions should undergo core needle or incisional biopsy. Care must be taken to orient the incisional biopsy in-line with any future operations, ensuring that the scar can be completely resected with the primary tumor. Ultrasound guidance or preoperative fiducial localization may help in planning the biopsy of non-palpable lesions. Patients with Ewing’s sarcoma, osteosarcoma, and some high-grade soft tissue sarcomas should be considered for induction therapy, while plasmacytomas should be treated with radiation alone. In addition, pulmonary function tests should be obtained on all patients prior to chest wall resection for risk-stratification.
As will be shown in this chapter, chest wall reconstruction is usually more complex than the resection itself and its requirements should be foreseen from the beginning and at every step of the excisional phase of the procedure.
Operative techniques
Preparation
Most patients undergoing chest wall resection and reconstruction should receive an epidural or paravertebral catheter for pain control, depending on the laterality and location of the surgery. Single-lung ventilation using a double-lumen endotracheal tube or bronchial blocker is advised for most cases. The positioning and draping of the patient will depend on the locations of the lesion and of any autologous tissue needed to cover the resultant defect. While sometimes the donor area can be prepped in advance, patient-repositioning during surgery while maintaining sterility, is not uncommon.
Chest wall resection
The overlying skin and soft-tissues are valuable assets for the reconstruction and should be preserved if the surgeon is certain of the absence of involvement with the lesion, thus performing the initial incision directly over the mass. As much subcutaneous tissue and muscle should be spared as possible, particularly muscles that are required for reconstruction, but never at the expense of a safe oncologic margin of 2–3 cm, circumferentially, around the tumor. The site(s) of previous biopsies should be excised en-bloc and, if the tumor is infiltrating the skin, an ellipse of dermis with at least 2–3 cm margins is resected on all sides.
For malignant primary chest wall tumors treated with radical intent, a 4-cm lateral margin and resection of a rib above and below the tumor is standard (1). The upper and lower intercostal spaces should be opened using electrocautery, allowing finger-palpation of the actual intrathoracic tumor margins. If the internal extent of the tumor is not clear, a thoracoscope can be inserted to help with localization. The intercostal muscle and neurovascular bundles can be sealed and cut using advanced bipolar energy or non-absorbable ligatures. The lateral rib margins are divided using guillotine rib shears. A frozen section analysis of relevant circumferential margins can be obtained during surgery and may be useful in decision-making if expanding the resection implies significant morbidity. However, this is only valid for soft tissue samples since the rib margins themselves must be decalcified prior to histologic examination. In any case, the resected specimen must be marked with threads on the sides to allow for accurate orientation by the pathologist. Pictures from several sides complement this information while showing the tissue before the fixation-induced retraction ensues.
In some cases, a partial or total sternectomy may be required to achieve adequate osseous margins (Figure 1). This involves performing costochondral release maneuvers to at least one rib space above and below the gross tumor margin. The internal mammary vessels should be identified and ligated. The dissection is generally performed from caudal to cephalad, as it is more convenient to lift the specimen up and dissect it off the underlying structures with electrocautery. If the manubrium is not involved, an oscillating saw can be used to divide the sternomanubrial junction. If necessary, the clavicular heads can be dissected in a sub-periosteal plane to avoid vascular injury and divided using an oscillating or Gigli saw.
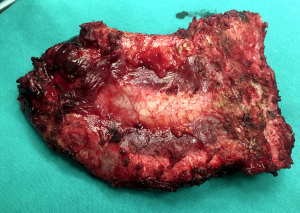
If the tumor is located posteriorly, the ribs may require disarticulation from the vertebrae. This involves identifying the joint between the posterior rib and transverse process and disrupting the three costotransverse attaching ligaments (superior, lateral and posterior), using a combination of electrocautery and blunt dissection. The remaining radiate ligament attaches the head of the rib to the superior and inferior vertebral bodies and should also be divided. A downward pressure by the surgeon’s assistant to the section of chest wall to be resected is helpful in the disarticulation maneuver.
Finally, in cases of tumor involvement of other underlying structures, similar appropriate clear margins must be obtained. For primary chest wall tumors, this includes achieving 2–3 cm margins on any affected non-vital adjacent organs, including lung, diaphragm, or potentially liver. This possibility should be thoroughly discussed with the patient prior to surgery.
Chest wall reconstruction
As a general principle, any defect larger than 5 cm in diameter or including more than 3 ribs should be reconstructed in order to avoid respiratory compromise from paradoxical motion of the chest wall. Reconstruction is also recommended when the defect extends lower than the 4th rib posteriorly, where the tip of the scapula is likely to become entrapped with the arm movements. There are two distinct technical steps in any reconstruction: (I) Restoring the structural and, if possible, functional rigidity of the chest wall; and (II) ensuring an adequate coverage of the defect with viable tissue (2). For the first step, there is a variety of implants and grafts which can be used.
A well-established technique involves the creation of a custom-shaped sandwich of methyl-methacrylate between two sheets of polypropylene mesh (Figure 2). An outline of the chest wall defect is marked on the polypropylene mesh and liquid methyl methacrylate (MMA) is poured over. The resin becomes rigid after curing, forming a cast that fills the chest wall defect. A second layer of mesh is then applied to create a sandwich (Figure 3). The rigid portion of the implant should be 1–2 cm smaller than the actual defect, allowing for a rim of mesh to be sutured circumferentially to the surrounding tissues. For this suture, heavy (No. 1–2) polypropylene thread is used, both in a pericostal (for the upper and lower ribs) and transcostal fashion (for the cut lateral rib margins). An alternative technique, which is particularly well-suited for sternal reconstruction, involves suturing a layer of mesh in place, filling it with MMA, and covering the defect with a second layer of mesh (Figure 4). If the polymerization of the MMA takes place in situ, additional care should be taken to avoid heat damage to the surrounding structures since this reaction is highly exothermic and temperatures in excess of 80 °C can be easily reached.
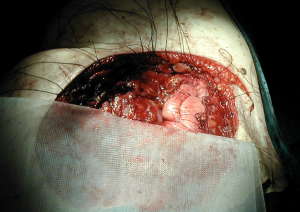
Polytetrafluoroethylene (PTFE) is another prosthetic material often used to reconstruct chest wall defects. A thick (2 mm) flexible sheet is anchored to the chest wall with heavy non-absorbable sutures. This mesh is very tightly stretched (“tight as a drum”) to provide rigidity to the chest wall and prevent both paradoxical respiratory motion and lung herniation. For this last purpose, the ideal placement is inside the level of the ribs (Figure 5). A bone drill or sharp towel clip work well for creating rib holes for fixation (Figure 6).
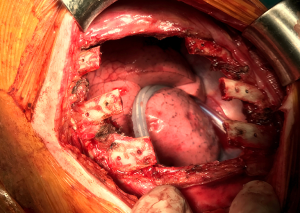
Concerning biological implants, a variety of cryopreserved homografts and allografts are also available in some centers and can be implanted using an identical technique (Figure 7). These grafts are usually hand milled at the time of reconstruction to cover a particular defect. In addition, any of these materials can be used in combination with bridging titanium plates to provide further structural stability. These plates can be fixed to the resected rib stumps using steel suture, cortical screws (Figure 8) or even simply clamping the terminal jaws which some of them include by design (Figures 9,10).
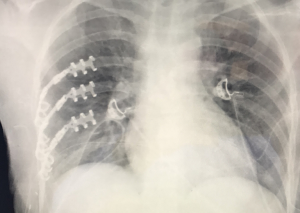
Chest wall reconstruction techniques have traditionally focused on restoring structural integrity by providing both rigidity and permanent covering. This is indeed enough for small to medium defects. For larger defects, or those involving sternocostal or vertebrocostal joints, biomechanical considerations apply, since maintaining the expandability and movement of the chest wall is essential to effective ventilation. In consequence, an optimal goal would be to restore as much functional integrity as possible. This could be achieved by incorporating some flexible parts and even “joints” with the attaching structures into the design of substitution implants. Metal implants have already been mentioned and can be flexed to a point during surgery, in order to accommodate different defects. This limited versatility and flexibility makes them less than ideal if we aim for a truly functional reconstruction (3).
A custom-made metal chest wall implant can be designed and molded or machine-milled but it is usually a time-consuming and costly process. More recently, an alternative based on 3D printing has been successfully applied in a few complex cases. This process involves several key steps: (I) Generating a high-resolution 3D reconstruction model of the chest wall, based on CT scans of the individual patient; (II) Planning the resection of bony structures on the 3D model, according to the size and location of the lesion and accounting for safe oncologic margins; (III) Designing a single or multiple-piece implant with the desired structural and biomechanical characteristics; (IV) Prototype 3D printing in hard plastic for surgeon handling prior to the procedure and refinement; (V) Final metal 3D printing of the implant, along with a cutting template (Figure 11), which the surgeon will find invaluable at the time of resection.
The design step is particularly crucial and has to involve both surgeons and biomedical engineers. It starts with precise functional and structural specifications and goes through several iterations until reaching an acceptable solution. Titanium is, again, the most commonly used metal for these implants, due to its excellent biocompatibility, lightness and durability. The degree and axes of flexibility of the different parts of the implant can be set by adjusting the thickness and folding of the metal (e.g., “Greek wave pattern”) (Figure 12).
The metal 3D printing phase is usually outsourced and employs two main technologies: electron beam melting (EBM) and laser beam sintering (LBS). Both are additive 3D printing methods in with titanium alloy powder is solidified into an extremely thin layer using highly focused energy, applied within a precise shape. This layer is melted over the previous one, in a high vacuum environment. The superposition of the fused layers along the Z-axis yields the final result, consisting on a single piece of solid titanium. The spatial resolution is excellent and will precisely follow the digital design. Current titanium 3D printing technologies allow for the construction of very complex shapes to fulfil functional demands, as well as osseointegrable surfaces or meshes to facilitate osteoblastic migration and proliferation.
From a practical point of view, the surgeon also has to carefully consider the best way to attach the 3D implant to the resection limits at the time of surgery. Already described methods such as cortical screws, foldable jaws o even simple steel sutures have been used so far. If we wish to remain faithful to functional principles, more creative solutions will be required for attachments to the spinal transverse processes or the sternum. Attachment of a metal implant to accessory respiratory muscles is still an unreached but desirable goal in selected cases.
In any case, these implants will usually be combined with underlying PTFE patches, which will provide sealing and separation of the pleural cavity (Figure 13). A viable and sufficiently thick layer of soft tissue over these metal implants, including muscle, is mandatory in order to prevent skin ulceration (4).
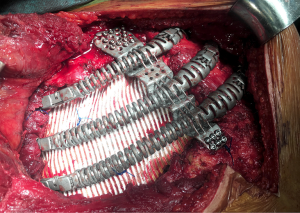
The second step in chest wall reconstruction is the coverage of the prosthesis with viable soft tissue. This is usually a muscular or myocutaneous graft, depending on the need for skin coverage. These grafts can be either pediculated (maintaining their original blood supply) or free vascularized grafts. Free vascularized grafts allow for greater versatility but require microsurgical vascular anastomoses and these procedures, often performed by plastic surgeons, fall off the scope of this chapter. The ability to harvest and transpose pediculated grafts, on the other hand, should be among the technical armamentarium of a thoracic surgeon, in case this resource was unexpectedly needed during scheduled oncologic surgery. The four most commonly used grafts are pectoralis muscle, rectus abdominis, latissimus dorsi and omental flaps.
Concerning the pectoralis major, it should be noted that it has a double blood supply: the pectoral branch of the thoracoacromial trunk of the subclavian artery and the internal mammary 2nd through 6th perforating branches. This wide and fairly thick muscle is well-suited to cover the sternum and other antero-superior chest wall defects, and is often mobilized bilaterally. The incision used to perform an anterior chest wall resection usually allows access to the pectoralis muscle. When a skin paddle is mobilized with the muscle, it should be tacked to the underlying pectoralis fascia (Figure 14). This prevents shearing of the perforating blood supply with mobilization, and the subsequent skin necrosis. Electrocautery is then used to separate the muscle from the underlying chest wall and overlying subcutaneous tissue, if needed (Figure 15). Scissor dissection should be used near the vascular pedicles to prevent injury. The thoracoacromial pedicle can often be palpated between the thumb and forefinger on the underside of the muscle, just medial to the coracoid process of the scapula (Figure 16). If the thoracoacromial vessels are inadequate, the pectoralis can also be used as a turn-over flap, vascularized by the internal mammary artery perforators. Division of the humeral and clavicular insertions allows extensive mobilization of the turn-over flap. Sparing 3–4 cm of muscle on the lateral aspect of the pectoralis muscle will leave the patient’s anterior axillary fold intact.
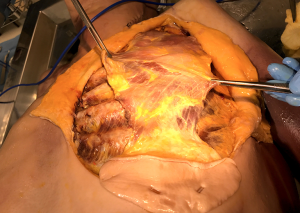
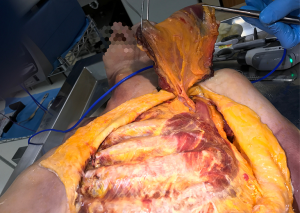
Most rectus abdominis flaps that are transposed onto chest wall defects derive their blood supply from the superior epigastric artery. The overlying skin paddle can be oriented transversely (TRAM) or vertically (VRAM). The size, location, and orientation of the harvested elliptical skin island are determined by the extent of chest wall defect and the ability to primarily close the donor site. After the skin incision, electrocautery is used to circumferentially dissect around the skin paddle down to the anterior rectus sheath, veering away from the skin to preserve perforating vessels. The anterior rectus sheath is then incised in a V-shape near the pubis and temporarily sutured to the overlying skin to avoid shearing of perforating vessels. The posterior rectus sheath should be dissected off the muscle and preserved, thus decreasing the risk of herniation. The rectus muscle is divided near the pubic insertion and, along with the overlying anterior rectus sheath and skin paddle, it is mobilized towards the xiphoid (Figure 17). The flap is swung under the epigastric skin and subcutaneous tissue and sutured in place to the chest wall defect. During initial mobilization, the umbilicus is mobilized and re-implanted after the anterior rectus sheath and abdominal skin are closed. It should be noted that both VRAM and TRAM flaps carry significant morbidity, mostly derived from delayed eventration, and other alternatives should be considered first, if possible.
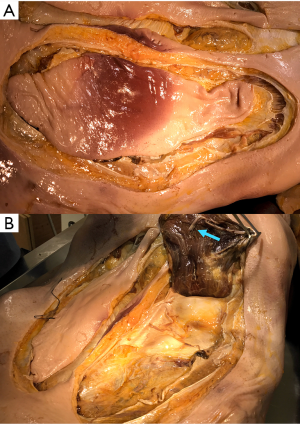
On the other hand, the latissimus dorsi flap is one of the muscles most commonly used for coverage of chest wall defects. It is a broad and flat muscle which is irrigated by the thoracodorsal artery, originating from the axillary artery. Virtually any skin island overlying the muscle can be harvested, limited only by the ability to close the donor site defect (Figures 18,19). If the latissimus cannot be accessed through the chest wall resection incision, a counter incision along the anterior aspect of the muscle can be fashioned. Electrocautery is used to mobilize the muscle from the underlying chest wall and surrounding subcutaneous tissues. The latissimus is differentiated from the underlying serratus muscle based on the longitudinal direction of its fibers. Dissection is carried caudally towards the iliac crest and posteriorly to the paraspinous muscles, where it is divided. As dissection continues cranially, the flap is lifted and care must be taken to preserve the thoracodorsal vascular pedicle. A few inches below the humeral insertion of the latissimus, the pulse can be palpated. Flap length can be gained by dividing the humeral insertion or by carefully removing the pedicle from its investing fascia with fine scissors (Figure 20).
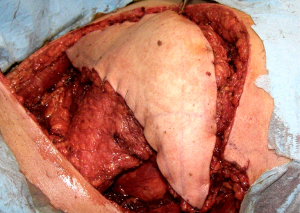
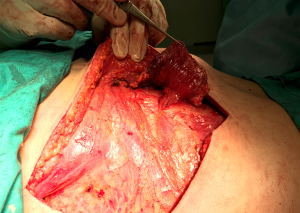
Finally, the omental flap, a vascularized fatty tissue, can reach nearly any location on the chest wall and is most often irrigated by the right gastroepiploic artery. Through a small upper midline laparotomy, the omentum should be freed from any bowel that it is adherent to and separated from the transverse colon. Small vessel ligation can be performed with silk ligatures or advanced bipolar energy. The short vessels between the greater curvature of the stomach and the gastroepiploic arch are then carefully divided and the left gastroepiploic artery is divided near the splenic hilum. If more length is needed, the omentum can be unfurled through selective division of internal vessels while preserving the vascular arcades (Figure 21). The omental flap is brought through the diaphragm or abdominal wall into place on the chest wall defect.
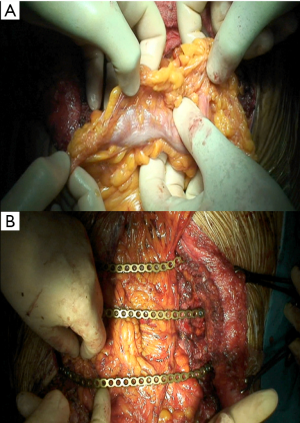
Comments
Tips and tricks
- Never compromise oncologic margins to preserve soft tissue required for chest wall reconstruction. Other options will be available;
- When harvesting myocutaneous flaps, suture the skin to the muscle fascia to avoid shearing delicate perforating vessels;
- Although electrocautery is used for most of the muscle flap mobilization, scissors should be used near the vascular pedicles to avoid thermal injury;
- It is important to leave an adequate number of soft tissue drains and not remove them until the drainage is less than 25 cc per day;
- If a PTFE graft becomes infected and the patient is not septic, immediate removal is not always indicated. If incision and drainage is performed, antibiotics are administered, and prosthesis removal is delayed for 6–8 weeks, enough scar tissue often forms to support the chest wall after the infected mesh is removed.
Caveats
Morbidity and mortality rates after chest wall resection and reconstruction range from 24–46% and 2–7%, respectively. The most common morbidities following chest wall resection and reconstruction relate to wound healing and pulmonary complications. Wound healing issues are more common in patients with advanced age, multiple re-do operations, combined prosthetic and bioprosthetic reconstruction, ulcerated tumors, and in those who have had soft tissue coverage with omentum. Although chest wall resection poses a considerable risk of developing postoperative pulmonary complications, without chest wall reconstruction, the incidence of flail chest and paradoxical motion, and resultant pulmonary complications, would likely be higher. In other words, reconstruction should always be carefully planned as part of the chest wall resection planning, as well as backup options.
Acknowledgements
None.
Footnote
Conflicts of Interest: The authors have no conflicts of interest to declare.
References
- McAfee MK, Pairolero PC, Bergstralh EJ, et al. Chondrosarcoma of the chest wall: factors affecting survival. Ann Thorac Surg 1985;40:535-41. [Crossref] [PubMed]
- Seder CW, Rocco G. Chest wall reconstruction after extended resection. J Thorac Dis 2016;8:S863-71. [Crossref] [PubMed]
- Rocco G. Chest wall resection and reconstruction according to the principles of biomimesis. Semin Thorac Cardiovasc Surg 2011;23:307-13. [Crossref] [PubMed]
- Moradiellos J, Amor S, Córdoba M, et al. Functional Chest Wall Reconstruction With a Biomechanical Three-Dimensionally Printed Implant. Ann Thorac Surg 2017;103:e389-e391. [Crossref] [PubMed]
Cite this article as: Moradiellos FJ, Seder CW, Rocco G. Chest wall resection and reconstruction. Shanghai Chest 2017;1:27.