The role of extracorporeal membrane oxygenation in thoracic surgery—a narrative review
Introduction
Background
Adequate ventilation is essential to maintain arterial oxygen saturations and remove excess carbon dioxide. The structures of interest in thoracic surgery play a key role in this process, and the shared area of intervention between surgeons and anaesthetists can make ventilation difficult or impossible using conventional methods. In recent years extracorporeal membrane oxygenation (ECMO) has been increasingly utilised to provide respiratory and haemodynamic support, whilst undertaking thoracic surgery.
ECMO has traditionally been used in an emergency setting to manage severe respiratory failure refractory to conventional medical therapy. Initially, rates of morbidity and mortality were high and early trials failed to show a benefit using this novel technology, so its use was limited to dire emergencies (1). Early ECMO circuits evolved from cardiopulmonary bypass (CPB) machines that were modified to remove the reservoir. Primitive oxygenators were not designed for long term use and caused significant systemic inflammatory reactions and damage to blood components. Over time, pump and oxygenator technology has improved and allowed prolonged use with fewer deleterious effects. Centrifugal pumps are magnetically levitated and cause less damage to blood components, and polymethyl-pentene (PMP) oxygenators can be used to provide long term ECMO support (2). These changes have led to a resurgence in the use of EMCO, most notably during the H1N1 influenza pandemic. This provided the opportunity to study the use of ECMO in large numbers of patients with acute respiratory distress syndrome (ARDS). Initial results were promising, with a landmark trial demonstrating improved outcomes when patients with severe respiratory failure were managed at specialised ECMO centres (3). However, a recent randomised controlled trial in a population of ARDS patients concluded that early initiation of ECMO was not associated with a significant reduction in mortality at 60 days (4). Despite this there has been a renewed interest in the application of ECMO and its use has increased significantly (5). As the use of ECMO has become more widely accepted, we have seen thoracic surgeons utilise this technology in range of elective and emergency situations, facilitating intervention for patients previously deemed too high risk or not suitable for surgery.
Objectives
This aim of this narrative review is to provide an overview of the development of ECMO, and how it can be applied in contemporary thoracic surgical practice. We present the following article in accordance with the Narrative Review reporting checklist (available at https://shc.amegroups.com/article/view/10.21037/shc-22-21/rc).
Methods
Library searches of PubMed® and EMBASE databases were undertaken to identify the literature for this narrative review. Search was undertaken on 5/1/22. Terms included were as follows: extra corporeal membrane oxygenation, ECMO, veno-arterial (VA)-ECMO, veno-venous (VV)-ECMO, thoracic surgery, lung transplantation, pneumonectomy, tracheobronchial surgery, mediastinal surgery, airway surgery, single lung ventilation. Timeframe for consideration was from origin to the date of the search. No restrictions were made on the basis of language. Search results were reviewed by the authors and the best evidence was included in this narrative review. Only published articles were included for review, unpublished articles or abstract only where relevant information was not accessible were excluded. All study types were considered for inclusion. Summary of the search strategy can be found in Table 1.
Table 1
Items | Specification |
---|---|
Date of search (specified to date, month and year) | January 5th, 2022 |
Databases and other sources searched | PubMed and EMBASE databases |
Search terms used (including MeSH and free text search terms and filters) | Search terms: (I) extra corporeal membrane oxygenation; (II) ECMO; (III) VA-ECMO; (IV) VV-ECMO; (V) thoracic surgery; (VI) lung transplantation; (VII) pneumonectomy; (VIII) tracheobronchial surgery; (IX) mediastinal surgery, (X) airway surgery, (XI) single lung ventilation |
Search strategy of PubMed database: (I) or (II) or (III) or (IV) and (V) or (VI) or (VII) or (VIII) or (IX) or (X). | |
Timeframe | From origin to January 5th 2022 |
Inclusion and exclusion criteria (study type, language restrictions etc.) | Inclusion criteria: (I) no language restrictions; (II) no article type restrictions |
Exclusion criteria: (I) un-published articles; (II) abstract only where relevant information was not accessible | |
Selection process (who conducted the selection, whether it was conducted independently, how consensus was obtained, etc.) | Search results were reviewed by the authors and the best evidence was included in this review. Consensus was obtained based on study design, and clinical experience of the topic being reviewed |
ECMO, extracorporeal membrane oxygenation.
Discussion
Advantages of ECMO
CPB was first used during thoracic surgery over sixty years ago and was the standard mode of extracorporeal support for some time (6). However, CPB has some distinct disadvantages. The air/blood interface introduced by the reservoir, and the large surface area of the circuit cause a significant systemic inflammatory response (SIRS) (7). There is activation of coagulation which requires significant heparinisation, with an activated clotting time (ACT) of at least 400 seconds. This greatly increases the risk of haemorrhage. The large prime volume causes significant haemodilution, causing further coagulopathy and electrolyte disturbance. Suctioned blood is returned to the venous reservoir, which in the instance of lung cancer surgery poses the risk of malignant cells entering the systemic circulation. CPB is also not suitable to continue respiratory or haemodynamic support into the postoperative period if required.
In contrast, modern ECMO circuits do not have a reservoir and utilise shorter lines, which reduces the circuit volume and limits the air/blood interface. This means there is less activation of coagulation therefore requiring much lower levels of heparinisation (ACT target 180–220 seconds). The reduced prime volume reduces haemodilution which in turn reduces coagulopathy. The reduced surface area and development of the materials used in circuit tubing reduces the induced SIRS response permitting more long term use (8,9). The differences between CPB and ECMO are summarised in Table 2.
Table 2
Cardiopulmonary bypass | Extracorporeal membrane oxygenation | |
---|---|---|
Prime volume | 1–2 L | 600 mL |
Pump type | Roller or centrifugal | Centrifugal |
Reservoir | Yes | No |
Activated clotting time (s) | >400 | 180–220 |
Duration of use | Short term | Short or long term |
Pre-operative support | No | Yes |
Post-operative support | No | Yes |
ECMO circuits are driven by magnetically levitated centrifugal pumps. A rotating magnet creates a centrifugal force, with the resulting pressure difference initiating flow through the circuit. This design causes less damage to blood components than the roller pumps sometimes used in CPB. As this design is dependent on a pressure gradient to function, it is influenced by preload and afterload and flow cannot be guaranteed by increasing the pump speed. In the event of sudden drop of inflow or increased resistance to outflow, respiratory or haemodynamic compromise can occur.
Oxygenator technology has improved drastically since the 1970s. Oxygenators are prone to fibrin and clot formation, which reduces their efficiency and increases the pressure required to maintain flow rates. Modern PMP oxygenators have a lower volume and more compact design and membranes that are less prone to leakage. This reduces the surface area, which causes less damage to blood components and a reduced the air/blood interface; allowing for more long term use before oxygenator failure occurs and reduced consumption of blood products (10).
Despite these advantages, ECMO circuits do have some limitations. Due to the lack of a reservoir, rapid reinfusion in the face of sudden volume loss is not possible, and blood products or drugs may need to be given by separate intravenous access. To counter this, attempts have been made to develop combined circuits that can be converted to CPB if required (11).
ECMO configurations
As described, early ECMO circuits evolved from CPB machines and were based on the same concept of venous drainage, extracorporeal oxygenation and arterial return. In the following decades other configurations have been developed to suit different clinical needs. They can be broadly divided into two main categories; veno-arterial (VA) and veno-venous (VV). The differences between these are summarised in Table 3.
Table 3
VV-ECMO | VA-ECMO | |
---|---|---|
CO2 removal | Low and high flow | Yes |
Oxygenation support | High flow | Yes |
Haemodynamic support | No | Yes |
Cannulation | Peripheral | Peripheral or central |
Risk of arterial injury | No | Yes |
Complications | Fewer comparted to VA | Increased risk of haemorrhagic andneurological |
ECMO, extracorporeal membrane oxygenation; VV, veno-venous; VA, veno-arterial.
VA-ECMO
VA-ECMO is the configuration most akin to CPB in that it drains deoxygenated blood from the venous system, and returns oxygenated blood to the arterial system. This provides full haemodynamic and respiratory support. Cannulation can be performed either centrally via the right atrium (RA) and aorta, peripherally via the femoral vein (FV) and femoral artery (FA) (Figure 1), or a combination of these. Central cannulation has the advantage of permitting larger bore cannula which allows higher flow rates to be achieved, however this comes with added risks as it necessitates an open thorax.
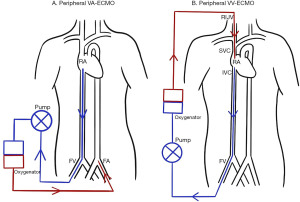
VA-ECMO (especially peripheral configurations) is associated with increased afterload and resulting left ventricle (LV) overload, LV dilatation and pulmonary oedema. Various percutaneous and surgical strategies have been developed to provide LV offloading whilst established on VA-ECMO. A common method is the use of an intra-aortic balloon pump (IABP). This improves LV ejection by decreasing afterload and also increases coronary perfusion. Various direct surgical vents can also be utilised, however these require access for insertion and removal (12). More recently, the use of percutaneous transaortic ventricular assist devices (Impella) have been used with good effect. These devices provide continuous flow from the LV into the aorta, reducing end diastolic LV volume and pulmonary oedema, as well as contributing to antegrade ECMO flow (13).
Limb ischaemia distal to the FA cannula can occur and an additional return cannula should be placed to provide limb perfusion if VA-ECMO is required for more than a few hours (14).
Depending on the flow rate and venous drainage, there is variable unloading of the right ventricle (RV) and much of the blood bypasses the pulmonary circulation. In the instance of peripheral cannulation via the FA, there is retrograde flow towards the thoracic aorta, and competing antegrade output from the LV. This causes a watershed area in the aorta where these opposing flows meet (15). The level at which this occurs depends on the pressure differential between the LV output and the ECMO flow and be anywhere from the aortic root to the distal thoracic aorta. This is of clinical significance as the oxygenation of the LV output is unknown and is dependent on pulmonary function, which is often impaired. This leads to the possibility of deoxygenated blood being delivered to the coronary, cerebral and upper limb circulations depending how far along the aorta the watershed occurs. In severe instances this can lead to North-South syndrome, characterised by upper body hypoxia and lower body hyperoxia (16). Arterial line monitoring and blood gas analysis from the right arm is essential to estimate the location of the watershed and assess for cerebral hypoxaemia. Management involves improving pulmonary function and treating underlying cardiogenic pulmonary oedema. As mentioned above, offloading the LV with additional venting strategies has also been described (12). If pulmonary function and oxygenation cannot be improved then alternative cannulation strategies must be employed. Adding a return cannula to the right axillary artery can help perfuse the innominate artery territories with oxygenated blood. Alternatively, an additional cannula that returns oxygenated blood into the venous system will improve the oxygenation of the LV output, this configuration is known as veno-arterial-veno (VAV) ECMO (17).
VV-ECMO
VV-ECMO is used when respiratory support is required without the need for haemodynamic support. Peripherally placed cannula drain venous blood, this is pumped through an oxygenator and returned to the venous circulation. Oxygenated blood is then delivered to the pulmonary circulation by the patients cardiac output. Although not having a direct effect on cardiac function, VV-ECMO can improve RV dysfunction caused by acute hypoxaemic respiratory failure by reducing RV preload and hypoxic pulmonary vasoconstriction (18).
VV-ECMO can be established using single lumen catheters, draining blood from the inferior vena cava (IVC) via the right FV, and returning it to the RA via the right internal jugular vein (Figure 1). With this setup the drainage cannula should be sited in the IVC below the hepatic vein, and the return cannula in the RA, just beyond the junction of the superior vena cava (SVC) and RA (19). Transoesophageal echocardiography (TOE) assessment of cannula position is recommended (20).
VV-ECMO can also be established using a single bi-caval dual lumen cannula. This is usually inserted via the right jugular vein. Deoxygenated blood is drained via ports in the SVC and IVC, and oxygenated blood is returned via a port sited in the RA facing towards the tricuspid valve (21). Ideal position of the dual lumen cannula is demonstrated in Figure 2. Dual lumen cannula insertion can be more challenging, and care must be taken to ensure the cannula passes through the RA into the IVC, and not through the tricuspid valve into the RV, which can cause catastrophic RV rupture. To mitigate these complications, placement should be visualised using TOE or preferably fluoroscopy (21). The single cannula also has the advantage of improving mobility, and facilitates the prone position if required (22).
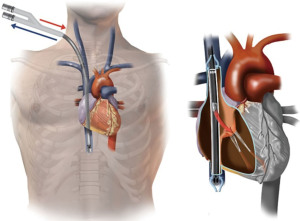
In VV-ECMO gas exchange is dependent on gradients across the oxygenator membrane. Oxygenation is controlled by changing the flow of blood through the oxygenator, and decarboxylation is controlled by the gas flow through the oxygenator (sweep) (23). Carbon dioxide can be removed at low flow rates by increasing the sweep, thus can be achieved with small cannula. High flow rates are required to ensure adequate oxygenation in the face of severe pulmonary dysfunction, and unventilated patients e.g., intraoperatively. Dual stage single cannula are recommended in these instances. Where the extent of lung injury permits, lower flow rates can be sufficient if traditional ventilation can also be utilised.
Patients who develop haemodynamic instability whilst established on VV-ECMO, can either be converted to a VA configuration, or alternatively converted to a veno-veno-arterial (VVA) configuration. VVA-ECMO utilises both venous cannula for drainage, and incorporates an additional cannula to return oxygenated blood to the arterial system, therefore providing haemodynamic as well as respiratory support (24,25).
Utilisation of ECMO in thoracic surgery
Tracheobronchial surgery
The tracheobronchial tree plays a key role in ventilation and surgical intervention on these structures poses unique challenges to surgeons and anaesthetists. There have been significant advances in anaesthetic techniques to try and overcome the challenges of maintaining ventilation whilst operating on the conducting airways. Tracheostomy cannula, cross field jet ventilation, direct cannulation of the distal trachea and small bore endotracheal tubes have all been employed. There are even cases of performing upper airway surgery on awake patients (26). Despite this, there are still circumstances where adequate ventilation cannot be maintained, and alternative strategies must be employed. A major advantage of ECMO in these situations is the ability to provide ventilatory support at a circulatory level, without the need for endotracheal intubation. Keeping the surgical site clear negates the difficulties of ventilating an open airway and facilitates challenging dissection and reconstruction.
A range of ECMO techniques have been utilised to manage this heterogeneous group of patients. Both VV and VA-ECMO have been used to support ventilation during carinal (27-29) and tracheal resections (30,31). Although VV-ECMO is the preferred method when possible, VA-ECMO has the advantage of proving haemodynamic support, which is often needed during more complex resections where retraction of the heart can cause haemodynamic compromise. VV-ECMO has also been utilised for endoscopic airway intervention, including endotracheal tumour resection and stent insertion/removal (20,32,33).
Iatrogenic tracheal injuries (ITI) are a rare but potentially life threatening complication of endotracheal intubation. Although a conservative approach is advised where possible, there are some cases which require surgical intervention, especially where life threatening respiratory compromise occurs (34). In these instances, VV-ECMO has been utilised to provide both pre-operative stabilisation as well as peri and post-operative ventilatory support (35-37). VV-ECMO has also been used to support repair of tracheoesophageal fistula secondary to chemoradiation (38) and oesophagectomy (39).
Thoracic emergencies
There are numerous emergency situations in thoracic surgery where ECMO has been employed for respiratory and haemodynamic support. The decision to initiate VV or VA ECMO in emergency situations is dictated by the primary need for respiratory or concomitant haemodynamic support.
VV-ECMO has been used for ventilatory support in critical airway obstruction secondary to foreign body aspiration (40) and malignancy (33). Pulmonary haemorrhage secondary to trauma (41), iatrogenic damage during surgery (42), and spontaneous bleeding (43) have all been managed with ECMO support. Traumatic airway rupture (44,45), and bronchopleural fistula (46) are further examples of challenging pathologies that have been successfully managed in the emergency setting with ECMO as an adjunct. VV and VA-ECMO have been used following cardiopulmonary arrest secondary to airway obstruction (20,47). Myocardial dysfunction is common in respiratory arrest hence the indication for VA-ECMO.
Inadequate single lung ventilation (SLV)
Thoracic surgery is often performed using SLV which can lead to difficulties in patients with underlying pulmonary compromise. Pre-operative pulmonary function testing can be used to predict intra-operative hypoxia and post-operative pulmonary reserve, however it is not always possible to perform these tests and inadequate ventilation can unexpectedly occur (48).
VV-ECMO has been used intra-operatively to support a wide range of procedures where reduced pulmonary reserve makes SLV unsafe; decortication of empyema (49), video-assisted thoracic surgery (VATS) bullectomy (50), VATS lobectomy, metastasectomy following previous lung resection (51), and bilateral lavage for alveolar proteinosis (52), are all described in the literature. More recently, VV-ECMO has been used to support robotic assisted lobectomy where severe emphysema prohibited SLV (53).
Another situation where SLV is impossible is patients undergoing further thoracic surgery following pneumonectomy. VV-ECMO support is described for contralateral lung resection for malignancy, bullectomy, and oesophageal resection post pneumonectomy (54-56).
Mediastinal pathology
Space occupying lesions in the anterior mediastinum can cause progressive compression of the RA, SVC, pulmonary artery and trachea; resulting in cardiorespiratory compromise. Surgical conditions and anaesthesia can exacerbate this, further reducing cardiac output. Both VV and VA-ECMO have been utilised to provide peri-operative support during resection of large mediastinal tumours (57). VA-ECMO may be preferred due to the additional haemodynamic support it offers.
Lung transplantation
Lung transplantation is often performed by thoracic surgeons, and ECMO is increasingly being used peri-operatively in this challenging patient cohort.
Patients awaiting lung transplantation often experience irreversible deterioration. In these instances ECMO can be utilised as a bridge to transplant, with the type of ECMO determined by the physiological need. Hypercapnic respiratory failure may be managed with low flow VV-ECMO. If there is severe hypoxia, full flow VV-ECMO with dual stage single lumen cannula may be required. If there is haemodynamic compromise, or severe pulmonary hypertension, VA-ECMO can be employed. In isolated pulmonary hypertension, a pumpless configuration using the PA-LA Novalung® has been developed. In this set-up, high pulmonary pressure drives flow into a low resistance oxygenator via a cannula inserted into the pulmonary artery. Arterial return is delivered via a cannula into the left atrium. This more compact system has the advantage of improving mobilisation, but requires a median sternotomy for initiation (58). Patients bridged to transplant with ECMO experience higher rates of morbidity and mortality, but can still achieve acceptable outcomes (59).
Due to the haemodynamic and respiratory compromise inherent to lung transplant surgery, intraoperative extracorporeal support is often required. Previously this was provided using CPB. However, there is growing evidence that patients supported with ECMO have better outcomes and reduced requirement for blood products when compared to CPB (60,61). This change is reflected in recent literature, with one study reporting that in the 51% of cases where extracorporeal support was required, ECMO was utilised in 80% of these (62). There are however some instances where CPB may still be required, for example, in the event of catastrophic bleeding. To overcome this problem some centres have developed hybrid ECMO circuits for lung transplantation that can be converted to CPB in emergency situations (11).
When established intraoperatively, central VA-ECMO is the usual configuration. Pre-operative VV-ECMO can be continued in some cases, or converted to central VA-ECMO if systemic hypotension or pulmonary hypertension are anticipated (63). Alternatively VV-ECMO can be converted to VVA-ECMO with the additional of an arterial cannula.
ECMO can be continued into the post-operative period if required. This is guided by haemodynamic status and graft function. A new ECMO circuit can also be initiated in the post-operative period to manage haemodynamic instability, primary graft dysfunction or reperfusion injury (64). Patients requiring VA-ECMO support into the post-operative period have a higher mortality rate when comparted to VV-ECMO (2).
Complications of ECMO
Despite the advantages ECMO can offer, it is an invasive procedure and complications are common. The nature of complex thoracic surgical patients requiring ECMO support means it can be hard to determine if complications are related to the underlying aetiology, or associated with ECMO related factors.
The most frequent complication encountered whilst established on ECMO is haemorrhage. The reported incidence of this varies widely (10–30%) (65,66) and it is more common in VA compared to VV-ECMO (34% and 17% respectively) (67). Occurrence of bleeding is associated with increased mortality (68), especially in the presence of intracranial haemorrhage (69). Bleeding risk is high due to variable levels of systemic heparinisation, SIRS response and coagulation activation, platelet dysfunction and clotting factor haemodilution (70).
Managing bleeding risk is complicated by the competing concerns of thrombotic events, which have been reported in approximately 15% of ECMO runs (71). Thrombi can form in the ECMO circuit, the consequences of which can be more serious in VA-ECMO as the return is directly into the patient’s arterial system. Clot formation can also occur in the patient, commonly venous cannulation is associated with deep vein thrombosis (72). Cardiac thrombosis can occur if adequate LV output is not maintained in the context of peripheral VA-ECMO.
Balancing the risk between bleeding and thrombosis requires careful anticoagulation monitoring and poses a considerable clinical challenge. Multiple diagnostic tests can be utilised to aid decision making including ACT, anti-factor Xa (anti-Xa), platelet count, activated partial thromboplastin time (aPTT) and international normalized ration (INR). ACT is used to monitor the anticoagulant effect of heparin, however, factors such as coagulopathy, platelet dysfunction and haemodilution can all prolong the ACT regardless of the heparin dose. This has raised concerns that ACT may not be a reliable test to guide heparin requirements for patients established on ECMO and there is growing evidence to suggest that ACT is poorly correlated to heparin dose in this context (73,74). Anti-Xa concentration measures the inhibition of factor Xa by heparin and is being increasingly used to monitor the effect of heparinisation. Notably, anti-Xa appears to be better correlated to heparin dose and may be a more suitable assay for anticoagulation monitoring (74).
VA-ECMO also carries additional risks associated with arterial cannulation. Other than bleeding described above, these include; embolization, aneurysm and dissection. Cannulation complications are of more concern in peripheral arterial cannulation due to the smaller vessels involved.
Neurological complications are frequent (10–20%) (75,76) and include haemorrhage, infarction, cerebral oedema and seizures. Other complications include infection, hypertension, renal failure, and gastrointestinal complications.
Due to the frequency and potential severity of these complications, the decision to initiate ECMO must not be taken lightly. Contraindications to the use of ECMO are summarised in Table 4. It is essential that these patients are managed in specialist centres under the guidance of an experienced multidisciplinary team.
Table 4
Items | Description |
---|---|
Absolute | • Irreversible aetiology |
• Limited life expectancy | |
• Unresolved malignancy | |
• Severe CNS injury | |
• Severe aortic regurgitation | |
• Unwitnessed cardiac arrest | |
• Peripheral arterial disease (peripheral VA-ECMO) | |
Relative | • Age |
• Duration of mechanical ventilation >7 days | |
• Objection to blood products | |
• Significant multi-organ failure | |
• Contraindication to anticoagulation |
ECMO, extracorporeal membrane oxygenation; CNS, central nervous system; VA, veno-arterial.
Complications relating to ECMO use have mostly been studied in critically unwell patient populations and emergency situations. These patients frequently have complex and severe underlying pathologies, and often longer duration of ECMO. As many complications relating to the use of ECMO are time dependent, it is not surprising the observed rates of complications are high. As described, many of the emerging uses of ECMO in thoracic surgery are taking place in elective patients for intraoperative support. When used in this context, ECMO is often only required to bridge the disruption to ventilation associated with the procedure and is usually weaned in the early post-operative period. ECMO runs lasting hours rather than days often have reduced heparin requirements, and reduced inflammatory and coagulation related issues, thus reducing the potential for bleeding and other time-dependent complications.
Conclusions
ECMO technology has developed significantly over recent decades and this has led to an increase in use outside its intended applications. We have provided an overview of how ECMO has been utilised in elective and emergency thoracic surgical patients.
With the exception of lung transplantation, the use of ECMO in thoracic surgery is far from routine and evidence is mostly limited to case reports and small series. Currently, larger randomised trials are unlikely to be feasible but are clearly warranted. The use of ECMO in these contexts is likely to continue to increase, and will provide opportunity for further study to better understand the possibilities and limitations of how ECMO can best be utilised in this challenging group of patients.
Acknowledgments
Funding: None.
Footnote
Provenance and Peer Review: This article was commissioned by the Guest Editors (Marcus Taylor and Felice Granato) for the series “Thoracic Surgery in High Risk Patients” published in Shanghai Chest. The article has undergone external peer review.
Reporting Checklist: The authors have completed the Narrative Review reporting checklist. Available at https://shc.amegroups.com/article/view/10.21037/shc-22-21/rc
Conflicts of Interest: Both authors have completed the ICMJE uniform disclosure form (available at https://shc.amegroups.com/article/view/10.21037/shc-22-21/coif). The series “Thoracic Surgery in High Risk Patients” was commissioned by the editorial office without any funding or sponsorship. The authors have no other conflicts of interest to declare.
Ethical Statement:
Open Access Statement: This is an Open Access article distributed in accordance with the Creative Commons Attribution-NonCommercial-NoDerivs 4.0 International License (CC BY-NC-ND 4.0), which permits the non-commercial replication and distribution of the article with the strict proviso that no changes or edits are made and the original work is properly cited (including links to both the formal publication through the relevant DOI and the license). See: https://creativecommons.org/licenses/by-nc-nd/4.0/.
References
- Zapol WM, Snider MT, Hill JD, et al. Extracorporeal membrane oxygenation in severe acute respiratory failure. A randomized prospective study. JAMA 1979;242:2193-6. [Crossref] [PubMed]
- Squiers JJ, Lima B, DiMaio JM. Contemporary extracorporeal membrane oxygenation therapy in adults: Fundamental principles and systematic review of the evidence. J Thorac Cardiovasc Surg 2016;152:20-32. [Crossref] [PubMed]
- Peek GJ, Mugford M, Tiruvoipati R, et al. Efficacy and economic assessment of conventional ventilatory support versus extracorporeal membrane oxygenation for severe adult respiratory failure (CESAR): a multicentre randomised controlled trial. Lancet 2009;374:1351-63. [Crossref] [PubMed]
- Combes A, Hajage D, Capellier G, et al. Extracorporeal Membrane Oxygenation for Severe Acute Respiratory Distress Syndrome. N Engl J Med 2018;378:1965-75. [Crossref] [PubMed]
- Thiagarajan RR, Barbaro RP, Rycus PT, et al. Extracorporeal Life Support Organization Registry International Report 2016. ASAIO J 2017;63:60-7. [Crossref] [PubMed]
- Woods FM, Neptune WB, Palatchi A. Resection of the carina and main-stem bronchi with the use of extracorporeal circulation. N Engl J Med 1961;264:492-4. [Crossref] [PubMed]
- Paparella D, Yau TM, Young E. Cardiopulmonary bypass induced inflammation: pathophysiology and treatment. An update. Eur J Cardiothorac Surg 2002;21:232-44. [Crossref] [PubMed]
- Machuca TN, Cypel M, Keshavjee S. Cardiopulmonary Bypass and Extracorporeal Life Support for Emergent Intraoperative Thoracic Situations. Thorac Surg Clin 2015;25:325-34. [Crossref] [PubMed]
- Ontaneda A, Annich GM. Novel Surfaces in Extracorporeal Membrane Oxygenation Circuits. Front Med (Lausanne) 2018;5:321. [Crossref] [PubMed]
- Khoshbin E, Roberts N, Harvey C, et al. Poly-methyl pentene oxygenators have improved gas exchange capability and reduced transfusion requirements in adult extracorporeal membrane oxygenation. ASAIO J 2005;51:281-7. [Crossref] [PubMed]
- Brazier A, Seville E, Hesford W, et al. The Wythenshawe Hybrid Circuit for lung transplantation: a previously undescribed circuit for lung transplant. Perfusion 2020;35:197-201. [Crossref] [PubMed]
- Rao P, Khalpey Z, Smith R, et al. Venoarterial Extracorporeal Membrane Oxygenation for Cardiogenic Shock and Cardiac Arrest. Circ Heart Fail 2018;11:e004905. [Crossref] [PubMed]
- Koeckert MS, Jorde UP, Naka Y, et al. Impella LP 2.5 for left ventricular unloading during venoarterial extracorporeal membrane oxygenation support. J Card Surg 2011;26:666-8. [Crossref] [PubMed]
- Makdisi G, Makdisi T, Wang IW. Use of distal perfusion in peripheral extracorporeal membrane oxygenation. Ann Transl Med 2017;5:103. [Crossref] [PubMed]
- Hoeper MM, Tudorache I, Kühn C, et al. Extracorporeal membrane oxygenation watershed. Circulation 2014;130:864-5. [Crossref] [PubMed]
- Lindholm JA. Cannulation for veno-venous extracorporeal membrane oxygenation. J Thorac Dis 2018;10:S606-12. [Crossref] [PubMed]
- Cakici M, Gumus F, Ozcinar E, et al. Controlled flow diversion in hybrid venoarterial-venous extracorporeal membrane oxygenation. Interact Cardiovasc Thorac Surg 2018;26:112-8. [Crossref] [PubMed]
- Grant C Jr, Richards JB, Frakes M, et al. ECMO and Right Ventricular Failure: Review of the Literature. J Intensive Care Med 2021;36:352-60. [Crossref] [PubMed]
- Douflé G, Ferguson ND. Monitoring during extracorporeal membrane oxygenation. Curr Opin Crit Care 2016;22:230-8. [Crossref] [PubMed]
- Ko M, dos Santos PR, Machuca TN, et al. Use of single-cannula venous-venous extracorporeal life support in the management of life-threatening airway obstruction. Ann Thorac Surg 2015;99:e63-5. [Crossref] [PubMed]
- Hirose H, Yamane K, Marhefka G, et al. Right ventricular rupture and tamponade caused by malposition of the Avalon cannula for venovenous extracorporeal membrane oxygenation. J Cardiothorac Surg 2012;7:36. [Crossref] [PubMed]
- Kuhl T, Michels G, Pfister R, et al. Comparison of the Avalon Dual-Lumen Cannula with Conventional Cannulation Technique for Venovenous Extracorporeal Membrane Oxygenation. Thorac Cardiovasc Surg 2015;63:653-62. [Crossref] [PubMed]
- Schmidt M, Tachon G, Devilliers C, et al. Blood oxygenation and decarboxylation determinants during venovenous ECMO for respiratory failure in adults. Intensive Care Med 2013;39:838-46. [Crossref] [PubMed]
- Yeo HJ, Jeon D, Kim YS, et al. Veno-veno-arterial extracorporeal membrane oxygenation treatment in patients with severe acute respiratory distress syndrome and septic shock. Crit Care 2016;20:28. [Crossref] [PubMed]
- Ius F, Sommer W, Tudorache I, et al. Veno-veno-arterial extracorporeal membrane oxygenation for respiratory failure with severe haemodynamic impairment: technique and early outcomes. Interact Cardiovasc Thorac Surg 2015;20:761-7. [Crossref] [PubMed]
- Macchiarini P, Rovira I, Ferrarello S. Awake upper airway surgery. Ann Thorac Surg 2010;89:387-90; discussion 390-1. [Crossref] [PubMed]
- Horita K, Itoh T, Furukawa K, et al. Carinal reconstruction under veno-venous bypass using a percutaneous cardiopulmonary bypass system. Thorac Cardiovasc Surg 1996;44:46-9. [Crossref] [PubMed]
- Keeyapaj W, Alfirevic A. Carinal resection using an airway exchange catheter-assisted venovenous ECMO technique. Can J Anaesth 2012;59:1075-6. [Crossref] [PubMed]
- Lang G, Ghanim B, Hötzenecker K, et al. Extracorporeal membrane oxygenation support for complex tracheo-bronchial procedures†. Eur J Cardiothorac Surg 2015;47:250-5; discussion 256. [Crossref] [PubMed]
- Liou JY, Chow LH, Chan KH, et al. Successful anesthetic management of a patient with thyroid carcinoma invading the trachea with tracheal obstruction, scheduled for total thyroidectomy. J Chin Med Assoc 2014;77:496-9. [Crossref] [PubMed]
- Kim CW, Kim DH, Son BS, et al. The Feasibility of Extracorporeal Membrane Oxygenation in the Variant Airway Problems. Ann Thorac Cardiovasc Surg 2015;21:517-22. [Crossref] [PubMed]
- Kim JJ, Moon SW, Kim YH, et al. Flexible bronchoscopic excision of a tracheal mass under extracorporeal membrane oxygenation. J Thorac Dis 2015;7:E54-7. [PubMed]
- Hong Y, Jo KW, Lyu J, et al. Use of venovenous extracorporeal membrane oxygenation in central airway obstruction to facilitate interventions leading to definitive airway security. J Crit Care 2013;28:669-74. [Crossref] [PubMed]
- Grewal HS, Dangayach NS, Ahmad U, et al. Treatment of Tracheobronchial Injuries: A Contemporary Review. Chest 2019;155:595-604. [Crossref] [PubMed]
- Hawkins RB, Thiele EL, Huffmyer J, et al. Extracorporeal membrane oxygenation for management of iatrogenic distal tracheal tear. JTCVS Tech 2020;4:389-91. [Crossref] [PubMed]
- Go PH, Pai A, Larson SB, et al. Repair of iatrogenic tracheal injury in acute respiratory failure with veno-venous extracorporeal membrane oxygenation. Perfusion 2021;36:100-2. [Crossref] [PubMed]
- Antonacci F, De Tisi C, Donadoni I, et al. Veno-venous ECMO during surgical repair of tracheal perforation: A case report. Int J Surg Case Rep 2018;42:64-6. [Crossref] [PubMed]
- van Drumpt AS, Kroon HM, Grüne F, et al. Surgery for a large tracheoesophageal fistula using extracorporeal membrane oxygenation. J Thorac Dis 2017;9:E735-8. [Crossref] [PubMed]
- Douin DJ, Tran TT. Bronchoesophageal Fistula Requiring Venovenous ECMO After Minimally Invasive Esophagectomy. J Cardiothorac Vasc Anesth 2020;34:2727-30. [Crossref] [PubMed]
- Park AH, Tunkel DE, Park E, et al. Management of complicated airway foreign body aspiration using extracorporeal membrane oxygenation (ECMO). Int J Pediatr Otorhinolaryngol 2014;78:2319-21. [Crossref] [PubMed]
- Park JM, Kim CW, Cho HM, et al. Induced airway obstruction under extracorporeal membrane oxygenation during treatment of life-threatening massive hemoptysis due to severe blunt chest trauma. J Thorac Dis 2014;6:E255-8. [PubMed]
- Fagundes Júnior AAP, Chaves RB, Santos ARD, et al. Massive hemoptysis successfully treated with extracorporeal membrane oxygenation and endobronchial thrombolysis. Rev Bras Ter Intensiva 2018;30:116-20. [Crossref] [PubMed]
- Abrams D, Agerstrand CL, Biscotti M, et al. Extracorporeal membrane oxygenation in the management of diffuse alveolar hemorrhage. ASAIO J 2015;61:216-8. [Crossref] [PubMed]
- Biscotti M, Gannon WD, Agerstrand C, et al. Awake Extracorporeal Membrane Oxygenation as Bridge to Lung Transplantation: A 9-Year Experience. Ann Thorac Surg 2017;104:412-9. [Crossref] [PubMed]
- Enomoto Y, Watanabe H, Nakao S, et al. Complete thoracic tracheal transection caused by blunt trauma. J Trauma 2011;71:1478. [Crossref] [PubMed]
- Al-Thani H, Ahmed K, Rizoli S, et al. Utility of extracorporeal membrane oxygenation (ECMO) in the management of traumatic tracheobronchial injuries: case series. J Surg Case Rep 2021;2021:rjab158. [Crossref] [PubMed]
- Willms DC, Mendez R, Norman V, et al. Emergency bedside extracorporeal membrane oxygenation for rescue of acute tracheal obstruction. Respir Care 2012;57:646-9. [Crossref] [PubMed]
- Slinger P, Suissa S, Adam J, et al. Predicting arterial oxygenation during one-lung ventilation with continuous positive airway pressure to the nonventilated lung. J Cardiothorac Anesth 1990;4:436-40. [Crossref] [PubMed]
- Brenner M, O'Connor JV, Scalea TM. Use of ECMO for resection of post-traumatic ruptured lung abscess with empyema. Ann Thorac Surg 2010;90:2039-41. [Crossref] [PubMed]
- Redwan B, Biancosino C, Giebel F, et al. Perioperative single-site veno-venous extracorporeal CO2 removal for minimally invasive giant bulla resection. Perfusion 2017;32:698-701. [Crossref] [PubMed]
- Redwan B, Ziegeler S, Freermann S, et al. Intraoperative veno-venous extracorporeal lung support in thoracic surgery: a single-centre experience. Interact Cardiovasc Thorac Surg 2015;21:766-72. [Crossref] [PubMed]
- Krecmerova M, Mosna F, Bicek V, et al. Extracorporeal membrane oxygenation to support repeated whole-lung lavage in a patient with pulmonary alveolar proteinosis in life threatening dyspnoe--a case report. BMC Anesthesiol 2015;15:173. [Crossref] [PubMed]
- Júnior ER, Terra RM, Guerreiro Cardoso PF, et al. Robotic lung volume reduction surgery with extracorporeal membrane oxygenation. Ann Thorac Surg 2022; [Epub ahead of print]. [PubMed]
- Gillon SA, Toufektzian L, Harrison-Phipps K, et al. Perioperative Extracorporeal Membrane Oxygenation to Facilitate Lung Resection After Contralateral Pneumonectomy. Ann Thorac Surg 2016;101:e71-3. [Crossref] [PubMed]
- Oey IF, Peek GJ, Firmin RK, et al. Post-pneumonectomy video-assisted thoracoscopic bullectomy using extra-corporeal membrane oxygenation. Eur J Cardiothorac Surg 2001;20:874-6. [Crossref] [PubMed]
- Schiff JH, Köninger J, Teschner J, et al. Veno-venous extracorporeal membrane oxygenation (ECMO) support during anaesthesia for oesophagectomy. Anaesthesia 2013;68:527-30. [Crossref] [PubMed]
- Leow L, Sampath HK, Yong KJ, et al. Rescue extracorporeal membrane oxygenation for massive anterior mediastinal masses. J Artif Organs 2021;24:450-7. [Crossref] [PubMed]
- Machuca TN, de Perrot M. Mechanical Support for the Failing Right Ventricle in Patients With Precapillary Pulmonary Hypertension. Circulation 2015;132:526-36. [Crossref] [PubMed]
- Hoetzenecker K, Donahoe L, Yeung JC, et al. Extracorporeal life support as a bridge to lung transplantation-experience of a high-volume transplant center. J Thorac Cardiovasc Surg 2018;155:1316-1328.e1. [Crossref] [PubMed]
- Biscotti M, Yang J, Sonett J, et al. Comparison of extracorporeal membrane oxygenation versus cardiopulmonary bypass for lung transplantation. J Thorac Cardiovasc Surg 2014;148:2410-5. [Crossref] [PubMed]
- Machuca TN, Collaud S, Mercier O, et al. Outcomes of intraoperative extracorporeal membrane oxygenation versus cardiopulmonary bypass for lung transplantation. J Thorac Cardiovasc Surg 2015;149:1152-7. [Crossref] [PubMed]
- Ruszel N, Kiełbowski K, Piotrowska M, et al. Central, peripheral ECMO or CPB? Comparsion between circulatory support methods used during lung transplantation. J Cardiothorac Surg 2021;16:341. [Crossref] [PubMed]
- Hashimoto K, Hoetzenecker K, Yeung JC, et al. Intraoperative extracorporeal support during lung transplantation in patients bridged with venovenous extracorporeal membrane oxygenation. J Heart Lung Transplant 2018;37:1418-24. [Crossref] [PubMed]
- Faccioli E, Terzi S, Pangoni A, et al. Extracorporeal membrane oxygenation in lung transplantation: Indications, techniques and results. World J Transplant 2021;11:290-302. [Crossref] [PubMed]
- Bartlett RH, Gattinoni L. Current status of extracorporeal life support (ECMO) for cardiopulmonary failure. Minerva Anestesiol 2010;76:534-40. [PubMed]
- Hemmila MR, Rowe SA, Boules TN, et al. Extracorporeal life support for severe acute respiratory distress syndrome in adults. Ann Surg 2004;240:595-605; discussion 605-7. [Crossref] [PubMed]
- Aubron C, Cheng AC, Pilcher D, et al. Factors associated with outcomes of patients on extracorporeal membrane oxygenation support: a 5-year cohort study. Crit Care 2013;17:R73. [Crossref] [PubMed]
- Aubron C, DePuydt J, Belon F, et al. Predictive factors of bleeding events in adults undergoing extracorporeal membrane oxygenation. Ann Intensive Care 2016;6:97. [Crossref] [PubMed]
- Australia and New Zealand Extracorporeal Membrane Oxygenation (ANZ ECMO) Influenza Investigators. Extracorporeal Membrane Oxygenation for 2009 Influenza A(H1N1) Acute Respiratory Distress Syndrome. JAMA 2009;302:1888-95. [Crossref] [PubMed]
- Murphy DA, Hockings LE, Andrews RK, et al. Extracorporeal membrane oxygenation-hemostatic complications. Transfus Med Rev 2015;29:90-101. [Crossref] [PubMed]
- Paden ML, Conrad SA, Rycus PT, et al. Extracorporeal Life Support Organization Registry Report 2012. ASAIO J 2013;59:202-10. [Crossref] [PubMed]
- Menaker J, Tabatabai A, Rector R, et al. Incidence of Cannula-Associated Deep Vein Thrombosis After Veno-Venous Extracorporeal Membrane Oxygenation. ASAIO J 2017;63:588-91. [Crossref] [PubMed]
- Atallah S, Liebl M, Fitousis K, et al. Evaluation of the activated clotting time and activated partial thromboplastin time for the monitoring of heparin in adult extracorporeal membrane oxygenation patients. Perfusion 2014;29:456-61. [Crossref] [PubMed]
- Delmas C, Jacquemin A, Vardon-Bounes F, et al. Anticoagulation Monitoring Under ECMO Support: A Comparative Study Between the Activated Coagulation Time and the Anti-Xa Activity Assay. J Intensive Care Med 2020;35:679-86. [Crossref] [PubMed]
- Lorusso R, Gelsomino S, Parise O, et al. Neurologic Injury in Adults Supported With Veno-Venous Extracorporeal Membrane Oxygenation for Respiratory Failure: Findings From the Extracorporeal Life Support Organization Database. Crit Care Med 2017;45:1389-97. [Crossref] [PubMed]
- Luyt CE, Bréchot N, Demondion P, et al. Brain injury during venovenous extracorporeal membrane oxygenation. Intensive Care Med 2016;42:897-907. [Crossref] [PubMed]
Cite this article as: Eadington T, Oommen K. The role of extracorporeal membrane oxygenation in thoracic surgery—a narrative review. Shanghai Chest 2022;6:22.